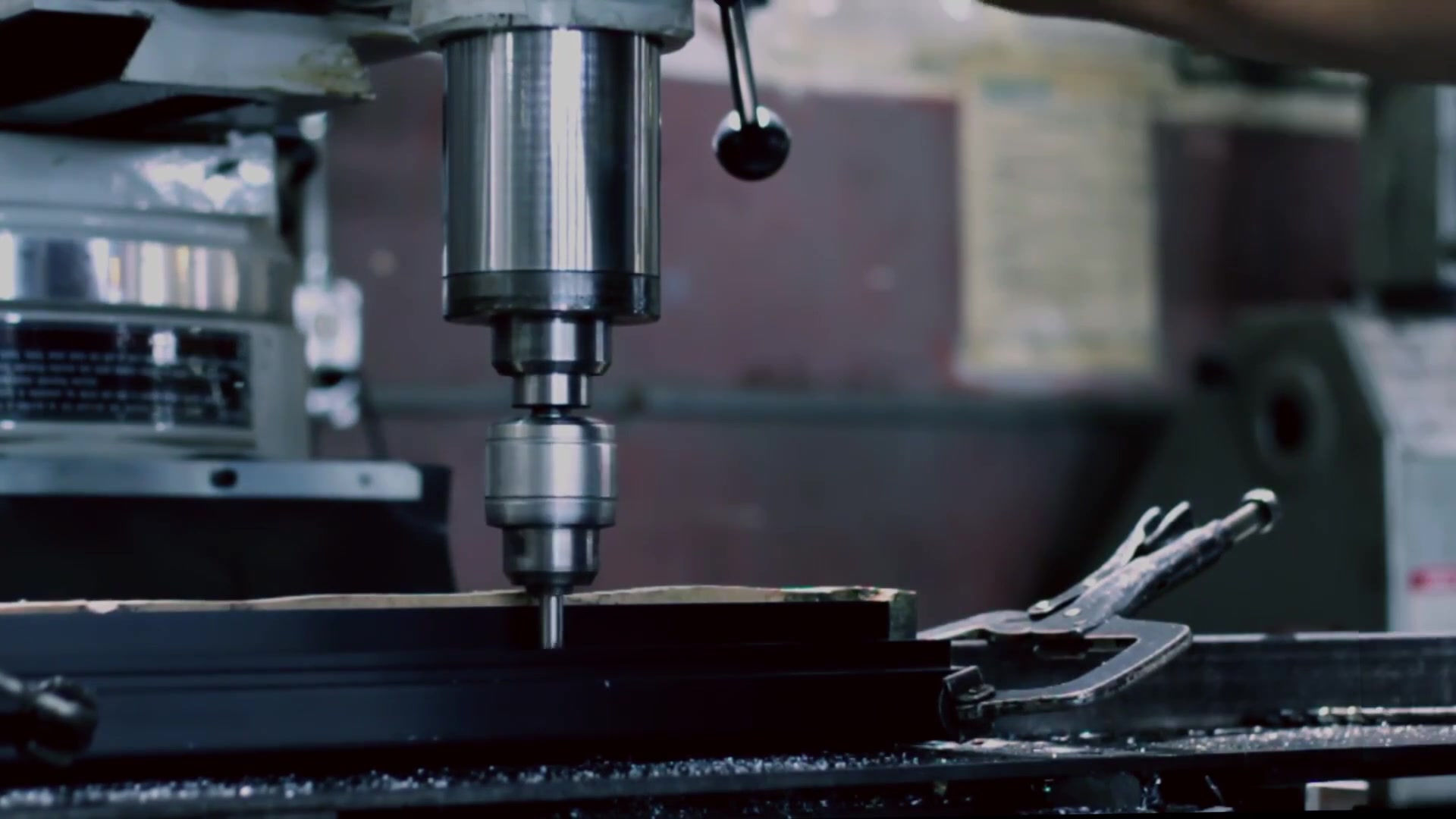
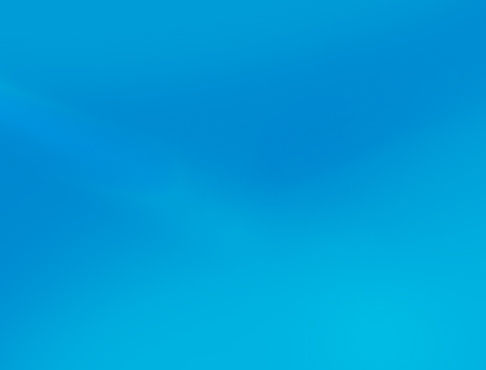
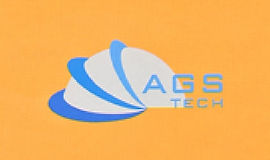
Global Custom Manufacturer, Integrator, Consolidator, Outsourcing Partner for a Wide Variety of Products & Services.
We are your one-stop source for manufacturing, fabrication, engineering, consolidation, integration, outsourcing of custom manufactured and off-shelf products & services. We also private label / white label your products with your brand name if you wish.
Choose your Language
-
Custom Manufacturing of Parts, Components, Assemblies, Finished Products, Machines and Industrial Equipment
-
Domestic & Global Contract Manufacturing
-
Manufacturing Outsourcing
-
Domestic, Global Procurement of Industrial Products
-
Private labeling / White Labeling your Products with your Brand Name
-
Product Finding & Locating Services
-
Global Design and Channel Partnership
-
Engineering Integration
-
Engineering Services
-
Global Consolidation, Warehousing, Logistics
With the term ELECTRONIC TESTER we refer to test equipment that is used primarily for testing, inspection and analysis of electrical and electronic components and systems. We offer the most popular ones in the industry:
POWER SUPPLIES & SIGNAL GENERATING DEVICES: POWER SUPPLY, SIGNAL GENERATOR, FREQUENCY SYNTHESIZER, FUNCTION GENERATOR, DIGITAL PATTERN GENERATOR, PULSE GENERATOR, SIGNAL INJECTOR
METERS: DIGITAL MULTIMETERS, LCR METER, EMF METER, CAPACITANCE METER, BRIDGE INSTRUMENT, CLAMP METER, GAUSSMETER / TESLAMETER/ MAGNETOMETER, GROUND RESISTANCE METER
ANALYZERS: OSCILLOSCOPES, LOGIC ANALYZER, SPECTRUM ANALYZER, PROTOCOL ANALYZER, VECTOR SIGNAL ANALYZER, TIME-DOMAIN REFLECTOMETER, SEMICONDUCTOR CURVE TRACER, NETWORK ANALYZER, PHASE ROTATION TESTER, FREQUENCY COUNTER
You can purchase brand new, refurbished or used test equipment from us at the most competitive discounted prices. Simply choose the product from the downloadable catalogs and let us know the product name, product code and relevant information and we will send you our quote. Download by clicking on highlighted text:
ANRITSU Electronic Measuring Instruments
KEYSIGHT Basic Automotive Test Products
KEYSIGHT Bench and Power Products
KEYSIGHT Network Analyzer Products
KEYSIGHT Signal Generation Solutions
KEYSIGHT Smart Bench Essentials Series Products
KEYSIGHT High-Volume Traffic Generator Products
KEYSIGHT Layer 4-7 Network Test Products
KEYSIGHT Layer 2-3 Network Test Products
KEYSIGHT Distribution Products Catalog
MEGGER Low Voltage Test Tools Catalog
MICROWAVE Flexible Cable Assembly
MICROWAVE and MILIMETER WAVE Test Accessories Brochure (Cable assemblies, VNA Test Assemblies, Mechanical Calibration Kits, RF Coaxial Adapters, Test Port Adapters, DC Blocks, NMD Connectors....etc.)
Private Label Hand Tools for Every Industry (This catalog contains a few electrical & electronic test instruments. We can private label these hand tools if you wish. In other words, we can put your company name, brand and label on them. This way you can promote your brand by reselling these to your customers.)
ROHDE SCHWARZ Benchtop Power Supplies
Ideal for labs and system racks, galvanic isolation, floating channels, constant voltage or current modes, protection functions, parallel and serial operation, low ripple/noise, remote sensing option
ROHDE SCHWARZ Test Equipment Catalog (Oscilloscopes, Power Supplies, Signal Generators, Handheld Analyzers, Spectrum Analyzers, Vector Network Analyzers, Meters & Counters)
TEKTRONIX Product Catalog for Test and Measurement Solutions
For details and other similar equipment, please visit our equipment website: http://www.sourceindustrialsupply.com
Let us briefly go over some of these equipment in everyday use throughout the industry:
The electrical power supplies we supply for metrology purposes are discrete, benchtop and stand-alone devices. The ADJUSTABLE REGULATED ELECTRICAL POWER SUPPLIES are some of the most popular ones, because their output values can be adjusted and their output voltage or current is maintained constant even if there are variations in input voltage or load current. ISOLATED POWER SUPPLIES have power outputs that are electrically independent of their power inputs. Depending on their power conversion method, there are LINEAR and SWITCHING POWER SUPPLIES. The linear power supplies process the input power directly with all their active power conversion components working in the linear regions, whereas the switching power supplies have components working predominantly in non-linear modes (such as transistors) and convert power to AC or DC pulses before processing. Switching power supplies are generally more efficient than linear supplies because they lose less power due to shorter times their components spend in the linear operating regions. Depending on application, a DC or AC power is used. Other popular devices are PROGRAMMABLE POWER SUPPLIES, where voltage, current or frequency can be remotely controlled through an analog input or digital interface such as an RS232 or GPIB. Many of them have an integral microcomputer to monitor and control the operations. Such instruments are essential for automated testing purposes. Some electronic power supplies use current limiting instead of cutting off power when overloaded. Electronic limiting is commonly used on lab bench type instruments. SIGNAL GENERATORS are another widely used instruments in lab and industry, generating repeating or non-repeating analog or digital signals. Alternatively they are also called FUNCTION GENERATORS, DIGITAL PATTERN GENERATORS or FREQUENCY GENERATORS. Function generators generate simple repetitive waveforms such as sine waves, step pulses, square & triangular and arbitrary waveforms. With Arbitrary waveform generators the user can generate arbitrary waveforms, within published limits of frequency range, accuracy, and output level. Unlike function generators, which are limited to a simple set of waveforms, an arbitrary waveform generator allows the user to specify a source waveform in a variety of different ways. RF and MICROWAVE SIGNAL GENERATORS are used for testing components, receivers and systems in applications such as cellular communications, WiFi, GPS, broadcasting, satellite communications and radars. RF signal generators generally work between a few kHz to 6 GHz, while microwave signal generators operate within a much wider frequency range, from less than 1 MHz to at least 20 GHz and even up to hundreds of GHz ranges using special hardware. RF and microwave signal generators can be classified further as analog or vector signal generators. AUDIO-FREQUENCY SIGNAL GENERATORS generate signals in the audio-frequency range and above. They have electronic lab applications checking of the frequency response of audio equipment. VECTOR SIGNAL GENERATORS, sometimes also referred to as DIGITAL SIGNAL GENERATORS are capable of generating digitally-modulated radio signals. Vector signal generators can generate signals based on industry standards such as GSM, W-CDMA (UMTS) and Wi-Fi (IEEE 802.11). LOGIC SIGNAL GENERATORS are also called DIGITAL PATTERN GENERATOR. These generators produce logic types of signals, that is logic 1s and 0s in the form of conventional voltage levels. Logic signal generators are used as stimulus sources for functional validation & testing of digital integrated circuits and embedded systems. The devices mentioned above are for general-purpose use. There are however many other signal generators designed for custom specific applications. A SIGNAL INJECTOR is a very useful and quick troubleshooting tool for signal tracing in a circuit. Technicians can determine the faulty stage of a device such as a radio receiver very quickly. The signal injector can be applied to the speaker output, and if the signal is audible one can move to the preceding stage of the circuit. In this case an audio amplifier, and if the injected signal is heard again one can move the signal injection up the stages of the circuit until the signal is no longer audible. This will serve the purpose of locating the location of the problem.
A MULTIMETER is an electronic measuring instrument combining several measurement functions in one unit. Generally, multimeters measure voltage, current, and resistance. Both digital and analog version are available. We offer portable hand-held multimeter units as well as laboratory-grade models with certified calibration. Modern multimeters can measure many parameters such as: Voltage (both AC / DC), in volts, Current (both AC / DC), in amperes, Resistance in ohms. Additionally, some multimeters measure: Capacitance in farads, Conductance in siemens, Decibels, Duty cycle as a percentage, Frequency in hertz, Inductance in henries, Temperature in degrees Celsius or Fahrenheit, using a temperature test probe. Some multimeters also include: Continuity tester; sounds when a circuit conducts, Diodes (measuring forward drop of diode junctions), Transistors (measuring current gain and other parameters), battery checking function, light level measuring function, acidity & Alkalinity (pH) measuring function and relative humidity measuring function. Modern multimeters are often digital. Modern digital multimeters often have an embedded computer to make them very powerful tools in metrology and testing. They include features such as::
•Auto-ranging, which selects the correct range for the quantity under test so that the most significant digits are shown.
•Auto-polarity for direct-current readings, shows if the applied voltage is positive or negative.
•Sample and hold, which will latch the most recent reading for examination after the instrument is removed from the circuit under test.
•Current-limited tests for voltage drop across semiconductor junctions. Even though not a replacement for a transistor tester, this feature of digital multimeters facilitates testing diodes and transistors.
•A bar graph representation of the quantity under test for better visualization of fast changes in measured values.
•A low-bandwidth oscilloscope.
•Automotive circuit testers with tests for automotive timing and dwell signals.
•Data acquisition feature to record maximum and minimum readings over a given period, and to take a number of samples at fixed intervals.
•A combined LCR meter.
Some multimeters can be interfaced with computers, while some can store measurements and upload them to a computer.
Yet another very useful tool, an LCR METER is a metrology instrument for measuring the inductance (L), capacitance (C), and resistance (R) of a component. The impedance is measured internally and converted for display to the corresponding capacitance or inductance value. Readings will be reasonably accurate if the capacitor or inductor under test does not have a significant resistive component of impedance. Advanced LCR meters measure true inductance and capacitance, and also the equivalent series resistance of capacitors and the Q factor of inductive components. The device under test is subjected to an AC voltage source and the meter measures the voltage across and the current through the tested device. From the ratio of voltage to current the meter can determine the impedance. The phase angle between the voltage and current is also measured in some instruments. In combination with the impedance, the equivalent capacitance or inductance, and resistance, of the device tested can be calculated and displayed. LCR meters have selectable test frequencies of 100 Hz, 120 Hz, 1 kHz, 10 kHz, and 100 kHz. Benchtop LCR meters typically have selectable test frequencies of more than 100 kHz. They often include possibilities to superimpose a DC voltage or current on the AC measuring signal. While some meters offer the possibility to externally supply these DC voltages or currents other devices supply them internally.
An EMF METER is a test & metrology instrument for measuring electromagnetic fields (EMF). Majority of them measure the electromagnetic radiation flux density (DC fields) or the change in an electromagnetic field over time (AC fields). There are single axis and tri-axis instrument versions. Single axis meters cost less than tri-axis meters, but take longer to complete a test because the meter only measures one dimension of the field. Single axis EMF meters have to be tilted and turned on all three axes to complete a measurement. On the other hand, tri-axis meters measure all three axes simultaneously, but are more expensive. An EMF meter can measure AC electromagnetic fields, which emanate from sources such as electrical wiring, while GAUSSMETERS / TESLAMETERS or MAGNETOMETERS measure DC fields emitted from sources where direct current is present. The majority of EMF meters are calibrated to measure 50 and 60 Hz alternating fields corresponding to the frequency of US and European mains electricity. There are other meters which can measure fields alternating at as low as 20 Hz. EMF measurements can be broadband across a wide range of frequencies or frequency selective monitoring only the frequency range of interest.
A CAPACITANCE METER is a test equipment used to measure capacitance of mostly discrete capacitors. Some meters display the capacitance only, whereas others also display leakage, equivalent series resistance, and inductance. Higher end test instruments use techniques such as inserting the capacitor-under-test into a bridge circuit. By varying the values of the other legs in the bridge so as to bring the bridge into balance, the value of the unknown capacitor is determined. This method ensures greater precision. The bridge may also be capable to measure series resistance and inductance. Capacitors over a range from picofarads to farads may be measured. Bridge circuits do not measure leakage current, but a DC bias voltage can be applied and the leakage measured directly. Many BRIDGE INSTRUMENTS can be connected to computers and data exchange be made to download readings or to control the bridge externally. Such bridge instruments aso offer go / no go testing for automation of tests in a fast paced production & quality control environment.
Yet, another test instrument, a CLAMP METER is an electrical tester combining a voltmeter with a clamp type current meter. Most modern versions of clamp meters are digital. Modern clamp meters have most of the basic functions of a Digital Multimeter, but with the added feature of a current transformer built into the product. When you clamp the instrument’s “jaws” around a conductor carrying a large ac current, that current is coupled through the jaws, similar to the iron core of a power transformer, and into a secondary winding which is connected across the shunt of the meter’s input, the principle of operation resembling much that of a transformer. A much smaller current is delivered to the meter’s input due to the ratio of the number of secondary windings to the number of primary windings wrapped around the core. The primary is represented by the one conductor around which the jaws are clamped. If the secondary has 1000 windings, then the secondary current is 1/1000 the current flowing in the primary, or in this case the conductor being measured. Thus, 1 amp of current in the conductor being measured would produce 0.001 amps of current at the input of the meter. With clamp meters much larger currents can be easily measured by increasing the number of turns in the secondary winding. As with most of our test equipment, advanced clamp meters offer logging capability. GROUND RESISTANCE TESTERS are used for testing the earth electrodes and the soil resistivity. The instrument requirements depend on the range of applications. Modern clamp-on ground testing instruments simplify ground loop testing and enable non-intrusive leakage current measurements.
Among the ANALYZERS we sell are OSCILLOSCOPES without doubt one of the most widely used equipment. An oscilloscope, also called an OSCILLOGRAPH, is a type of electronic test instrument that allows observation of constantly varying signal voltages as a two-dimensional plot of one or more signals as a function of time. Non-electrical signals like sound and vibration can also be converted to voltages and displayed on oscilloscopes. Oscilloscopes are used to observe the change of an electrical signal over time, the voltage and time describe a shape which is continuously graphed against a calibrated scale. Observation and analysis of the waveform reveals us properties such as amplitude, frequency, time interval, rise time, and distortion. Oscilloscopes can be adjusted so that repetitive signals can be observed as a continuous shape on the screen. Many oscilloscopes have storage function that allows single events to be captured by the instrument and displayed for a relatively long time. This allows us to observe events too fast to be directly perceptible. Modern oscilloscopes are lightweight, compact and portable instruments. There are also miniature battery-powered instruments for field service applications. Laboratory grade oscilloscopes are generally bench-top devices. There is a vast variety of probes and input cables for use with oscilloscopes. Please contact us in case you need advice about which one to use in your application. Oscilloscopes with two vertical inputs are called dual-trace oscilloscopes. Using a single-beam CRT, they multiplex the inputs, usually switching between them fast enough to display two traces apparently at once. There are also oscilloscopes with more traces; four inputs are common among these. Some multi-trace oscilloscopes use the external trigger input as an optional vertical input, and some have third and fourth channels with only minimal controls. Modern oscilloscopes have several inputs for voltages, and thus can be used to plot one varying voltage versus another. This is used for example for graphing I-V curves (current versus voltage characteristics) for components such as diodes. For high frequencies and with fast digital signals the bandwidth of the vertical amplifiers and sampling rate must be high enough. For-general purpose use a bandwidth of at least 100 MHz is usually sufficient. A much lower bandwidth is sufficient for audio-frequency applications only. Useful range of sweeping is from one second to 100 nanoseconds, with appropriate triggering and sweep delay. A well-designed, stable, trigger circuit is required for a steady display. The quality of the trigger circuit is key for good oscilloscopes. Another key selection criteria is the sample memory depth and sample rate. Basic level modern DSOs now have 1MB or more of sample memory per channel. Often this sample memory is shared between channels, and can sometimes only be fully available at lower sample rates. At the highest sample rates the memory may be limited to a few 10's of KB. Any modern ''real-time'' sample rate DSO will have typically 5-10 times the input bandwidth in sample rate. So a 100 MHz bandwidth DSO would have 500 Ms/s - 1 Gs/s sample rate. Greatly increased sample rates have largely eliminated the display of incorrect signals that was sometimes present in the first generation of digital scopes. Most modern oscilloscopes provide one or more external interfaces or buses such as GPIB, Ethernet, serial port, and USB to allow remote instrument control by external software. Here is a list of different oscilloscope types:
CATHODE RAY OSCILLOSCOPE
DUAL-BEAM OSCILLOSCOPE
ANALOG STORAGE OSCILLOSCOPE
DIGITAL OSCILLOSCOPES
MIXED-SIGNAL OSCILLOSCOPES
HANDHELD OSCILLOSCOPES
PC-BASED OSCILLOSCOPES
A LOGIC ANALYZER is an instrument that captures and displays multiple signals from a digital system or digital circuit. A logic analyzer may convert the captured data into timing diagrams, protocol decodes, state machine traces, assembly language. Logic Analyzers have advanced triggering capabilities, and are useful when the user needs to see the timing relationships between many signals in a digital system. MODULAR LOGIC ANALYZERS consist of both a chassis or mainframe and logic analyzer modules. The chassis or mainframe contains the display, controls, control computer, and multiple slots into which the data-capturing hardware is installed. Each module has a specific number of channels, and multiple modules can be combined to obtain a very high channel count. The ability to combine multiple modules to obtain a high channel count and the generally higher performance of modular logic analyzers makes them more expensive. For the very high end modular logic analyzers, the users may need to provide their own host PC or purchase an embedded controller compatible with the system. PORTABLE LOGIC ANALYZERS integrate everything into a single package, with options installed at the factory. They generally have lower performance than modular ones, but are economical metrology tools for general purpose debugging. In PC-BASED LOGIC ANALYZERS, the hardware connects to a computer through a USB or Ethernet connection and relays the captured signals to the software on the computer. These devices are generally much smaller and less expensive because they make use of a personal computer’s existing keyboard, display and CPU. Logic analyzers can be triggered on a complicated sequence of digital events, then capture large amounts of digital data from the systems under test. Today specialized connectors are in use. The evolution of logic analyzer probes has led to a common footprint that multiple vendors support, which provides added freedom to end users: Connectorless technology offered as several vendor-specific trade names such as Compression Probing; Soft Touch; D-Max is being used. These probes provide a durable, reliable mechanical and electrical connection between the probe and the circuit board.
A SPECTRUM ANALYZER measures the magnitude of an input signal versus frequency within the full frequency range of the instrument. The primary use is to measure the power of the spectrum of signals. There are optical and acoustical spectrum analyzers as well, but here we will discuss only electronic analyzers that measure and analyze electrical input signals. The spectra obtained from electrical signals provides us information about frequency, power, harmonics, bandwidth…etc. The frequency is displayed on the horizonal axis and the signal amplitude on the vertical. Spectrum analyzers are widely used in the electronics industry for the analyses of the frequency spectrum of radio frequency, RF and audio signals. Looking at the spectrum of a signal we are able to reveal elements of the signal, and the performance of the circuit producing them. Spectrum analyzers are able to make a large variety of measurements. Looking at the methods used to obtain the spectrum of a signal we can categorize the spectrum analyzer types.
- A SWEPT-TUNED SPECTRUM ANALYZER uses a superheterodyne receiver to down-convert a portion of the input signal spectrum (using a voltage-controlled oscillator and a mixer) to the center frequency of a band-pass filter. With a superheterodyne architecture, the voltage-controlled oscillator is swept through a range of frequencies, taking advantage of the full frequency range of the instrument. Swept-tuned spectrum analyzers are descended from radio receivers. Therefore swept-tuned analyzers are either tuned-filter analyzers (analogous to a TRF radio) or superheterodyne analyzers. In fact, in their simplest form, you could think of a swept-tuned spectrum analyzer as a frequency-selective voltmeter with a frequency range that is tuned (swept) automatically. It is essentially a frequency-selective, peak-responding voltmeter calibrated to display the rms value of a sine wave. The spectrum analyzer can show the individual frequency components that make up a complex signal. However it does not provide phase information, only magnitude information. Modern swept-tuned analyzers (superheterodyne analyzers, in particular) are precision devices that can make a wide variety of measurements. However, they are primarily used to measure steady-state, or repetitive, signals because they can't evaluate all frequencies in a given span simultaneously. The ability to evaluate all frequencies simultaneously is possible with only the real-time analyzers.
- REAL-TIME SPECTRUM ANALYZERS: A FFT SPECTRUM ANALYZER computes the discrete Fourier transform (DFT), a mathematical process that transforms a waveform into the components of its frequency spectrum, of the input signal. The Fourier or FFT spectrum analyzer is another real-time spectrum analyzer implementation. The Fourier analyzer uses digital signal processing to sample the input signal and convert it to the frequency domain. This conversion is done using the Fast Fourier Transform (FFT). The FFT is an implementation of the Discrete Fourier Transform, the math algorithm used for transforming data from the time domain to the frequency domain. Another type of real-time spectrum analyzers, namely the PARALLEL FILTER ANALYZERS combine several bandpass filters, each with a different bandpass frequency. Each filter remains connected to the input at all times. After an initial settling time, the parallel-filter analyzer can instantaneously detect and display all signals within the analyzer's measurement range. Therefore, the parallel-filter analyzer provides real-time signal analysis. Parallel-filter analyzer is fast, it measures transient and time-variant signals. However, the frequency resolution of a parallel-filter analyzer is much lower than most swept-tuned analyzers, because the resolution is determined by the width of the bandpass filters. To get fine resolution over a large frequency range, you would need many many individual filters, making it costly and complex. This is why most parallel-filter analyzers, except the simplest ones in the market are expensive.
- VECTOR SIGNAL ANALYSIS (VSA) : In the past, swept-tuned and superheterodyne spectrum analyzers covered wide frequency ranges from audio, thru microwave, to millimeter frequencies. In addition, digital signal processing (DSP) intensive fast Fourier transform (FFT) analyzers provided high-resolution spectrum and network analysis, but were limited to low frequencies due to the limits of analog-to-digital conversion and signal processing technologies. Today's wide-bandwidth, vector-modulated, time-varying signals benefit greatly from the capabilities of FFT analysis and other DSP techniques. Vector signal analyzers combine superheterodyne technology with high speed ADC's and other DSP technologies to offer fast high-resolution spectrum measurements, demodulation, and advanced time-domain analysis. The VSA is especially useful for characterizing complex signals such as burst, transient, or modulated signals used in communications, video, broadcast, sonar and ultrasound imaging applications.
According to form factors, spectrum analyzers are grouped as benchtop, portable, handheld and networked. Benchtop models are useful for applications where the spectrum analyzer can be plugged into AC power,such as in a lab environment or manufacturing area. Bench top spectrum analyzers generally offer better performance and specifications than the portable or handheld versions. However they are generally heavier and have several fans for cooling. Some BENCHTOP SPECTRUM ANALYZERS offer optional battery packs, allowing them to be used away from a mains outlet. Those are referred to as a PORTABLE SPECTRUM ANALYZERS. Portable models are useful for applications where the spectrum analyzer needs to be taken outside to make measurements or carried while in use. A good portable spectrum analyzer is expected to offer optional battery-powered operation to allow the user to work in places without power outlets, a clearly viewable display to allow the screen to be read in bright sunlight, darkness or dusty conditions, light weight. HANDHELD SPECTRUM ANALYZERS are useful for applications where the spectrum analyzer needs to be very light and small. Handheld analyzers offer a limited capability as compared to larger systems. Advantages of handheld spectrum analyzers are however their very low power consumption, battery-powered operation while in the field to allow the user to move freely outside, very small size & light weight. Finally, NETWORKED SPECTRUM ANALYZERS do not include a display and they are designed to enable a new class of geographically-distributed spectrum monitoring and analysis applications. The key attribute is the ability to connect the analyzer to a network and monitor such devices across a network. While many spectrum analyzers have an Ethernet port for control, they typically lack efficient data transfer mechanisms and are too bulky and/or expensive to be deployed in such a distributed manner. The distributed nature of such devices enable geo-location of transmitters, spectrum monitoring for dynamic spectrum access and many other such applications. These devices are able to synchronize data captures across a network of analyzers and enable Network-efficient data transfer for a low cost.
A PROTOCOL ANALYZER is a tool incorporating hardware and/or software used to capture and analyze signals and data traffic over a communication channel. Protocol analyzers are mostly used for measuring performance and troubleshooting. They connect to the network to calculate key performance indicators to monitor the network and speed-up troubleshooting activities. A NETWORK PROTOCOL ANALYZER is a vital part of a network administrator's toolkit. Network protocol analysis is used to monitor the health of network communications. To find out why a network device is functioning in a certain way, administrators use a protocol analyzer to sniff the traffic and expose the data and protocols that pass along the wire. Network protocol analyzers are used to
- Troubleshoot hard-to-solve problems
- Detect and identify malicious software / malware. Work with an Intrusion Detection System or a honeypot.
- Gather information, such as baseline traffic patterns and network-utilization metrics
- Identify unused protocols so that you can remove them from the network
- Generate traffic for penetration testing
- Eavesdrop on traffic (e.g., locate unauthorized Instant Messaging traffic or wireless Access Points)
A TIME-DOMAIN REFLECTOMETER (TDR) is an instrument that uses time-domain reflectometry to characterize and locate faults in metallic cables such as twisted pair wires and coaxial cables, connectors, printed circuit boards,….etc. Time-Domain Reflectometers measure reflections along a conductor. In order to measure them, the TDR transmits an incident signal onto the conductor and looks at its reflections. If the conductor is of a uniform impedance and is properly terminated, then there will be no reflections and the remaining incident signal will be absorbed at the far end by the termination. However, if there is an impedance variation somewhere, then some of the incident signal will be reflected back to the source. The reflections will have the same shape as the incident signal, but their sign and magnitude depend on the change in impedance level. If there is a step increase in the impedance, then the reflection will have the same sign as the incident signal and if there is a step decrease in impedance, the reflection will have the opposite sign. The reflections are measured at the output/input of the Time-Domain Reflectometer and displayed as a function of time. Alternatively, the display can show the transmission and reflections as a function of cable length because the speed of signal propagation is almost constant for a given transmission medium. TDRs can be used to analyze cable impedances and lengths, connector and splice losses and locations. TDR impedance measurements provide designers the opportunity to perform signal integrity analysis of system interconnects and accurately predict the digital system performance. TDR measurements are widely used in board characterization work. A circuit board designer can determine the characteristic impedances of board traces, compute accurate models for board components, and predict board performance more accurately. There are many other areas of application for time-domain reflectometers.
A SEMICONDUCTOR CURVE TRACER is a test equipment used to analyze the characteristics of discrete semiconductor devices such as diodes, transistors, and thyristors. The instrument is based on oscilloscope, but contains also voltage and current sources that can be used to stimulate the device under test. A swept voltage is applied to two terminals of the device under test, and the amount of current that the device permits to flow at each voltage is measured. A graph called V-I (voltage versus current) is displayed on the oscilloscope screen. Configuration includes the maximum voltage applied, the polarity of the voltage applied (including the automatic application of both positive and negative polarities), and the resistance inserted in series with the device. For two terminal devices like diodes, this is sufficient to fully characterize the device. The curve tracer can display all of the interesting parameters such as the diode's forward voltage, reverse leakage current, reverse breakdown voltage,…etc. Three-terminal devices such as transistors and FETs also use a connection to the control terminal of the device being tested such as the Base or Gate terminal. For transistors and other current based devices, the base or other control terminal current is stepped. For field effect transistors (FETs), a stepped voltage is used instead of a stepped current. By sweeping the voltage through the configured range of main terminal voltages, for each voltage step of the control signal, a group of V-I curves is generated automatically. This group of curves makes it very easy to determine the gain of a transistor, or the trigger voltage of a thyristor or TRIAC. Modern semiconductor curve tracers offer many attractive features such as intuitive Windows based user interfaces, I-V, C-V and pulse generation, and pulse I-V, application libraries included for every technology…etc.
PHASE ROTATION TESTER / INDICATOR: These are compact and rugged test instruments to identify phase sequence on three-phase systems and open/de-energized phases. They are ideal for installing rotating machinery, motors and for checking generator output. Among the applications are the identification of proper phase sequences, detection of missing wire phases, determination of proper connections for rotating machinery, detection of live circuits.
A FREQUENCY COUNTER is a test instrument that is used for measuring frequency. Frequency counters generally use a counter which accumulates the number of events occurring within a specific period of time. If the event to be counted is in electronic form, simple interfacing to the instrument is all that is needed. Signals of higher complexity may need some conditioning to make them suitable for counting. Most frequency counters have some form of amplifier, filtering and shaping circuitry at the input. Digital signal processing, sensitivity control and hysteresis are other techniques to improve performance. Other types of periodic events that are not inherently electronic in nature will need to be converted using transducers. RF frequency counters operate on the same principles as lower frequency counters. They have more range before overflow. For very high microwave frequencies, many designs use a high-speed prescaler to bring the signal frequency down to a point where normal digital circuitry can operate. Microwave frequency counters can measure frequencies up to almost 100 GHz. Above these high frequencies the signal to be measured is combined in a mixer with the signal from a local oscillator, producing a signal at the difference frequency, which is low enough for direct measurement. Popular interfaces on frequency counters are RS232, USB, GPIB and Ethernet similar to other modern instruments. In addition to sending measurement results, a counter can notify the user when user-defined measurement limits are exceeded.
For details and other similar equipment, please visit our equipment website: http://www.sourceindustrialsupply.com